Each year, millions of central venous catheters and other indwelling devices are inserted in various healthcare settings worldwide. These widely used devices predispose the emergence of central line-associated bloodstream infections (CLABSI), which contribute to prolonged hospital stays.
Furthermore, these infections may require a complex therapeutic strategy, which may have limited efficacy. One of the most important predisposing factors to CLABSIs is the biofilm-forming ability of pathogenic microorganisms. The bacterial and/or fungal cells adhere to these abiotic surfaces and develop a thick, three-dimensional, polysaccharide-based matrix, which provides resistance to various environmental factors, the immune response, and the majority of antimicrobial drugs. Moreover, the dispersed cells from these sessile communities may be a continuous source of life-threatening systemic infection.
Based on data from the Centers for Disease Control and Prevention (CDC), a remarkable decrease in rates of pathogen-specific CLABSI rates in intensive care units was observed between 2011 and 2017. However, this trend could not be detected in cases of Candida-related central line-associated infections. In addition, these microbes were the most common CLABSI-related pathogens in 2017. Based on current medical guidelines, immediate catheter replacement must be applied in cases of confirmed Candida-associated catheter-related infections. Nevertheless, catheter replacement is not feasible for all patients, especially for those who have limited venous access.
Recently published alternative therapies for the treatment of Candida-related CLABSI include high-dose therapy with licensed antifungal drugs, combination-based therapeutic approaches, and antifungal lock therapy. Experimental data have revealed the therapeutic potential of echinocandins and amphotericin B formulations against fungal biofilms, both in traditional therapies and in the abovementioned alternative approaches such as antifungal lock therapy. However, the number of resistant fungal infections has been steadily increasing, and new potentially multi-resistant fungal species have emerged in clinical practice, such as Candida auris, multiazole-resistant Aspergillus isolates or Lomentospora prolificans, causing further challenges for therapy.
Despite the relatively widely used antibacterial lock protocols, unfortunately there is no approved antifungal lock therapy in clinical practice. Lock therapy uses prolonged instillation of a solution containing high concentrations of antimicrobial compounds within an infected catheter in order to sterilise it and remove intraluminal biofilms. A relatively novel and innovative alternative therapeutic approach against Candida biofilms – which can be used as part of a potential antifungal lock protocol – is the disruption of the fungal quorum-sensing process. Quorum sensing is a major mechanism of microbial cell–cell communication, acting as a population density-dependent stimulus response mediated by hormone-like low-molecular-weight secreted molecules called quorum-sensing molecules. To date, four major fungal quorum-sensing molecules have been described, including farnesol, tyrosol, phenylethanol and tryptophol, which each have a remarkable effect on the regulation of morphogenesis.
In the last decade, the number of studies dealing with the effect of farnesol against Candida species has steadily increased, supporting the comprehensive understanding of the potential antifungal effect exerted by this compound. At physiological concentrations, farnesol has no significant inhibitory effect if hyphae development or biofilm formation have already begun. However, several studies demonstrate that farnesol at supraphysiological concentrations can cause biofilm degradation, particularly increasing the rate of apoptotic fungal cells. Furthermore, it can enhance the activity of several traditional antifungal agents such as azoles, amphotericin B formulations and echinocandins. Moreover, it may cause resistance reversion, especially in the case of azoles. The antifungal activity exerted by farnesol can be explained by the extensive production of reactive oxygen species and the inhibition of fungal survival strategies. Farnesol has an amphiphilic nature, allowing its integration into fungal cell membranes, potentially influencing the membrane integrity. Furthermore, it inhibits manganese, zinc transport and iron metabolism, and increases the fungal intracellular copper content – thereby disrupting intracellular metal homeostasis. Interestingly, fungal metabolism is modulated towards beta-oxidation following farnesol exposure.
Tyrosol is a relatively understudied and underappreciated molecule compared with farnesol, with regard to its antifungal activity and synergistic effect with antifungal drugs. Previous studies have described a potential antifungal effect similar to that of farnesol at supraphysiological concentrations; furthermore, the compound enhanced the activity of certain traditional antifungal drugs against biofilms. Based on deep molecular analysis, tyrosol exposure may be able to enhance oxidative stress, and inhibit growth, ribosome biogenesis and virulence in the case of Candida cells. Surprisingly, metabolism is modulated towards glycolysis and ethanol fermentation, unlike on exposure to farnesol.
In summary, the possible introduction of this novel type of therapy into clinical practice even in lock therapy is unlikely to occur in the near future. To date, the extent of the available data focusing on fungal quorum sensing is limited. Nevertheless, there may be huge potential in this innovative therapeutic approach against fungal biofilms that would be a mistake to leave untapped.
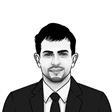
No comments yet